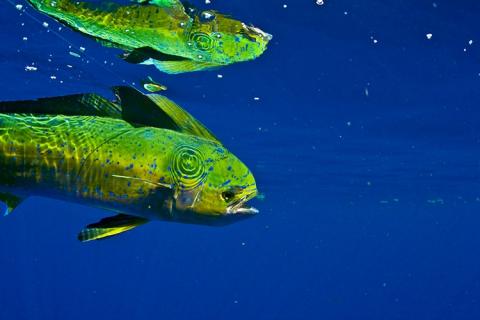
The underwater world abounds with noise. Gamefish, through an intricate network of receptors, utilize this sound to move within their environment, detect threats, and locate and feed on prey. But without visible ears how do fish hear? Is sound really that important of a consideration that we should be taking notice? Like anything in angling, understanding the traits and biological makeup of gamefish help us get inside a fish's head. And that can only mean better days of fishing in the future.
![]() |
Located inside the skull, a bass is estimated to have 20,000 or more sensory hair cells. |
The underwater world abounds with noise. Gamefish, through an intricate network of receptors, utilize this sound to move within their environment, detect threats, and locate and feed on prey. But without visible ears how do fish hear? Is sound really that important of a consideration that we should be taking notice? Like anything in angling, understanding the traits and biological makeup of gamefish help us get inside a fish's head. And that can only mean better days of fishing in the future.
Taking a Look Underwater
The properties of water are drastically different than those of air and not for the most obvious of reasons. Sound becomes a density disturbance once below water and is able to travel at a speed independent of the sound frequency. It is transmitted almost five times faster through water (4,920 feet per second) and may be interpreted over a greater distance. Traveling at just under a mile a second, these multi-directional disturbances can be blocked by structure and cover or may be reflected due to chemical factors, such as a thermocline. Increases in frequency have a direct correlation to the weakening of sounds.
In his book, Knowing Bass — The Scientific Approach to Catching More Fish, Dr. Keith Jones explains the acoustics of water:
"Any object moving in water produces two acoustic effects: localized water currents and pressure waves. To understand the difference, let's take the example of a baitfish swimming. With each stroke of its head, the fish pushes water molecules away from its body. This produces localized flow, or miniature currents, that move outward in the surrounding water. The water is pulled along the backside of the head and tail, again causing currents in the form of miniature swirls or vortices. Besides pushing water, the moving tail and head also crowd the neighboring water molecules together. These molecules, in turn, crowd against their neighbors, and they against their neighbors, and so on. The result is a series of compression waves that originate at the baitfish and spread rapidly outward in everexpanding arcs. The first of these physical effects, the localized current, is known as a hydrodynamic disturbance; the second, the pressure wave, is typically referred to as true sound. Both types of acoustical disturbances are inherent to sound fields. You don't get one without the other. However, each has distinctly different effects on nearby predatory fish."
Factors affecting the speed of sound and the distance it travels include water temperature, salinity and overall depth. Dense water allows sound to travel faster, with saltwater reigning supreme over fresh.
Sound spreads throughout the water column in an arc — meeting with the surface at the top and the bottom composition down below as it travels outwards. The surface of the water is an excellent reflector of sound waves, and once it reaches this pinnacle, the waves are bounced back into the water column itself. Add some turbulence to the water, such as those caused by waves, and the sound itself becomes scattered. This also holds true when sound bounces off sedimentary bottoms, such as mud or sand. Rocky bottoms, however, reflect sound much better but are capable of scattering sound due to their rough surfaces.
As depth increases in a lake, sound intensity does also. In addition to this, sound frequency can be lost completely the shallower the water gets, as every sound frequency requires a minimum depth to be heard. Low-frequency sounds propagate poorly in shallow water.
Detecting Sound
Unlike humans, gamefish have two sensors for detecting acoustical disturbances — the inner ear and lateral line. These two systems work independently of each other to hear and feel particle motion and pressure changes, and they are able to correlate and send signals to various parts of the brain to initiate a reaction or function.
The lateral line is comprised of two major divisions, including the cephalic system — which is located on the head — and one found along the body trunk called the lateralis. Fish use each in varying ways, as the structure makeup and nerve branches differ greatly.
"The cephalic system is a network of highly branched canals spread over the head and face of the fish, especially around the eyes, across the top of the head, beneath the jaw, and along the front portion of the gill cover," writes Jones. "The lateralis system consists of a single long canal on either side of the trunk, starting just behind the eyes and extending to the tail. The head canals are partially or completely housed in the underlying bone, whereas the main trunk canal is constructed from specialized scales overlying the skin."
Pores are spaced evenly along these canals, allowing water to flow freely along the canal itself. Found between these pores and within the canal is a gelatin mass called a cupula. Beneath this mass is a sensory patch — named the neuromast — which plays host to a unique variety of hair cells that interpret overall senses. These hair cells protrude into the cupula and are extremely sensitive to its every move. Separate nerves service the trunk and face of a fish and both branches eventually enter the rear of the brain together with their respective information.
A human ear is comprised of three distinct divisions. The ear of a fish has only one: the inner ear. Housed within the skull and beneath the skin, the ear itself is not visible. Three canals or arches are responsible for controlling equilibrium, and each are connected to an open sac called the labyrinth. Three sensory patches are located within this sac, and much like the lateral line, each contains hair cells.
Found on top of each of these three patches is a calcified ear stone, or otolith. Each of these organs contains thousands of sensory hair cells (a bass is estimated to have 20,000 or more) and is connected to nerve fibers that relay the messages to the brain.
Both the lateral line and inner ear are distance receptors. If a fish is situated a distance from the sound source, it can still pick up and digest acoustic signals. However, the lateral line is only considered effective within two body lengths or less from the source. Go beyond this and the lateral line dramatically loses its range, leaving the inner ears responsible for the majority of the far off sound detection. Lateral line sensors are thought to detect only low-frequency oscillations, as these are necessary to trigger lateral line nerve cells. This is ideal as most aquatic biological sounds are focused in the lower frequency ranges.
Using Sound to Our Advantage
The environment of a fish is often a good indicator of how important sound is. As the vision of a fish decreases — such as in stained or murky water and during low-light periods — detecting sound and vibration becomes paramount to success when feeding.
![]() |
Using a rattle-type bait in thick weeds or dense cover increases chances of a fish honing in on your bait. |
Wil Wegman, a successful tournament angler and seminar host, offered this insight into sound detection: "Muddy or off-colored water are prime conditions in my books for noisy lures. Understanding that when the sense of sight of a fish is diminished it is forced to rely more on its other senses (like sound and vibration, which is typically picked up through it's lateral line) in order to capitalize on feeding opportunities. It is my belief as well that over time fish that continually reside in murky/dirty water conditions have become genetically programmed to become optimal predators within the living quarters they are dealt, and sound detection becomes a primary tool for survival."
Charles Sim, an Ottawa-area tournament angler and competitor south of the border agrees: "If I'm fishing dirty water, I will use a bait with rattles or a lure that gives off a tonne of vibration. The same holds true for working thick weed or 'junk' — I'll use a rattle-type bait in order to help the fish locate my lure."
It makes sense that the thicker the cover you are working, the less of a reliance vision plays for a fish honing in on your bait. Typical scenarios would include thick, weed-choked bays, expansive sections of lily pads and slop patches.
"When working within the nastiest cover imaginable, I will use a lure with built-in rattles," Wegman said. "Here, I am competing — or at least my bait is — with all weeds or other 'junk' around and I will pull out all the stops to get a fish to take notice, or 'hear' my bait."
Are there certain environmental conditions were sound can become a detriment? Definitely so. "Bluebird sky, mid-day, little or no wind and relatively clear water — under these conditions, please don't make me use my favorite rattling bait!" explained Wegman. "When there is little competition from other environmental factors influencing how well a fish can see or 'feel' a bait within the water column, I basically shy away from lures with rattles."
As discussed earlier, low-frequency sounds propagate poorly in shallow water since the wavelength is longer than the water depth. Although these frequencies may be lost in the shallows, fish may be able to detect them in deeper water. Just because that noisy crankbait came up empty-handed in 3 feet of water doesn't mean it will do so poorly in 30 feet.
'Big' Jim McLaughlin offered this insight into making that first cast: "I am a firm believer that you always start with a noisy lure first and then maybe go to something that is of a stealthier approach. Always fish aggressively to start, and that means noise too."
Background noise can also have an affect on fish detecting your bait. Depending on the frequency or loudness of this secondary sound, competing with background noise to be heard may mean a switch to a lure with a different frequency or one that is optimally louder. The most common of these external factors would be boat traffic, beaches with human activity and environmental sounds, such as wave action and rain.
"It makes sense for lure sound patterns to emulate those of natural prey. This is where softbaits tend to shine over hardbaits," Jones writes in his book. "As white-noise generators, hardbaits do not put out sounds anywhere close to those of natural prey. The subtle soft plastic baits, on the other hand, as they slide and slink along, generate vibrations that come much close to natural sounds. While natural sounds may work, the most attractive sounds will be those that best match a fish's sensory tuning, whether the sound is natural or artificial. A fish doesn't know the difference. It has no concept of natural, only whether an object matches what it is designed to perceive."
Sound can be a complex concept when dealing with the underwater world. Science continues to study the inner workings of gamefish, but as you can see, hearing is an important part of a fish's life — and a detail we can all utilize when experimenting with sound out on the lake.
- 5172 views